Specific physical constraints on land
Transparency is common in organisms living in water but it is really rare in organisms living on land. Why so? Several physical factors may contribute to explaining this pattern.
- The difference in refraction indexes. A refraction index (ri) measures the velocity of light in a medium. The higher the refraction index, the lower the speed of light. At an interface between two media, the percentage of light reflected by the surface increases as the difference in refraction index values between the two media increases. A biological tissue (ranging from ri=1.35 for cytoplasm or wax to 1.55 for proteins) is more different in refraction index when surrounded by air (ri=1) than when surrounded by water (ri=1.34). As a consequence, the surface reflections of a transparent organism in air are 10-fold to 2000-fold greater than its surface reflections in seawater. A transparent organism is thus more likely to be betrayed by reflections in air than in water and this constraint has likely been a selective force that has favoured various mechanisms that would limit surface reflections in air.
- Gravity. On land, there is less supportive forces than in water, which may impose on organisms living on land self-sustained tissues, and this may imply light-absorbing material (bone, shell…).
- High levels of UV radiations. Pigments absorb UV radiations that are detrimental for cells and DNA. UV radiations are much higher in air than in water, and more abundant in water near the surface than deeper below. Only rare pigments absorb UV without absorbing human-visible wavelengths (but mycosporine-like amino acids do). Being transparent may be too costly in terms of UV protection for organisms living on land. Being pigmented can be an advantage as pigments can contribute to cryptic colorations.
This may explain why on land, transparency is mostly restricted to insect wings.
How is transparency achieved in Lepidoptera (butterflies and moths)?
Among the 124 extant Lepidoptera families, at least 31 families comprise species that have evolved fully or partially transparent wings. Except few species with clear wings, these families comprise opaque species. This shows that transparency has evolved several times independently in these lineages. Looking at 123 species with transparent or translucent wings from these 31 families (Gomez et al 2021) shows a high diversity in the structures that produce transparency in wings. Transparency can arise from a nude membrane with no scales, a membrane covered with highly modified piliform scales (long and thin), a membrane covered with lamellar scales of various shapes (other than piliform) or a membrane covered with both lamellar and piliform scales. These microstructures on the wing membrane can be erect or flat on the wing membrane and they can be coloured (any colour) or they may be at least partially transparent. In some species, piliform and lamellar scales can also be combined (both of the same insertion and colour) and be present in the same transparent patch .
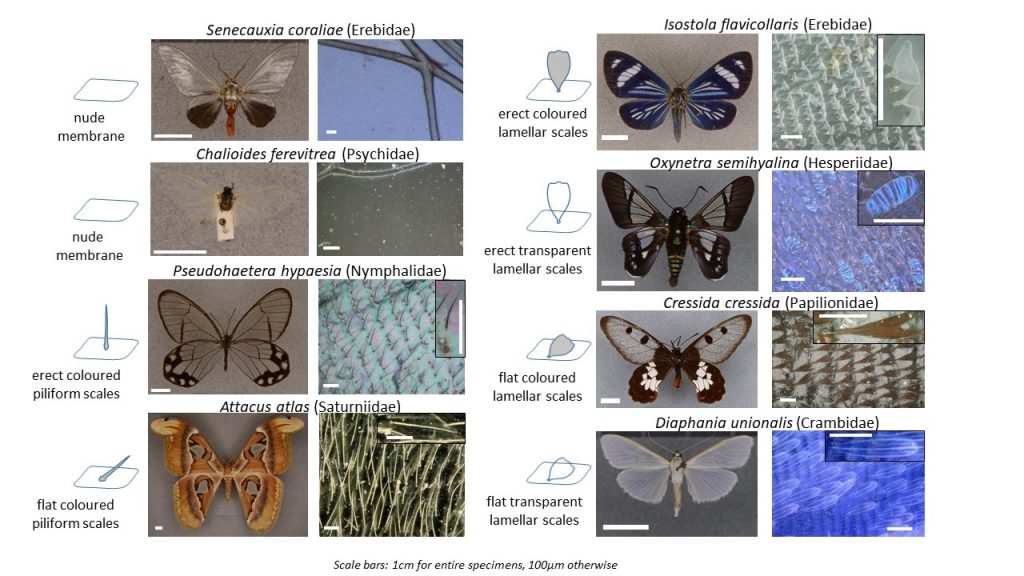
Developmental pathways leading to transparent wings
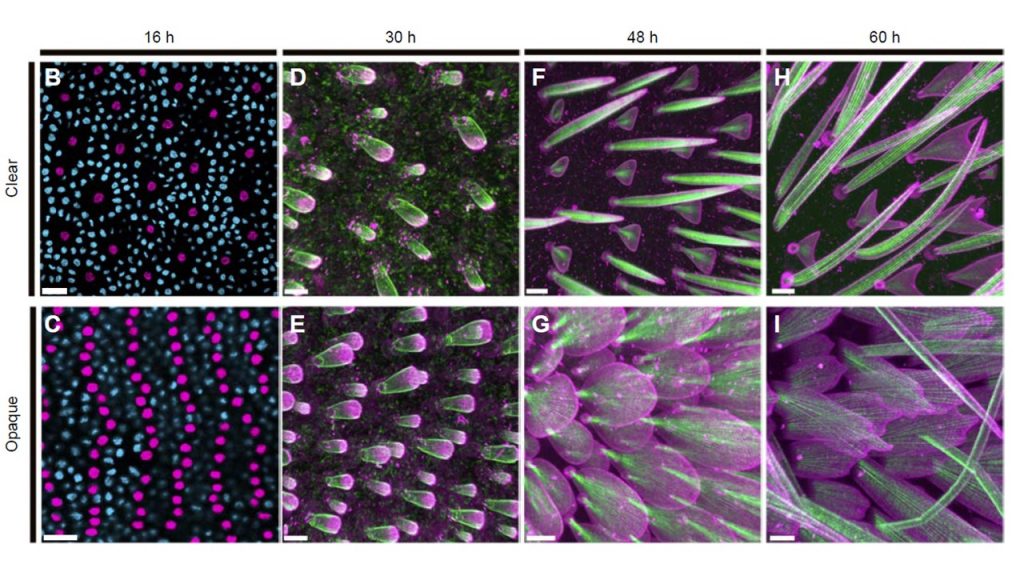
We applied confocal and electron microscopy to create a developmental time series in the glasswing butterfly, Greta oto, comparing clear and opaque wing regions (Pomerantz et al 2021). We found that during early wing development (16h after pupal formation), scale precursor cell density was reduced in transparent regions. Cytoskeletal organization during scale growth differed between thin, bristle-like scale morphologies within transparent regions and flat, round scale morphologies within opaque regions. Please notice that in lamellar scales, scales are fully developed before being partially digested following the F-actin intracellular pattern. Serrations appear and forked cells are formed.
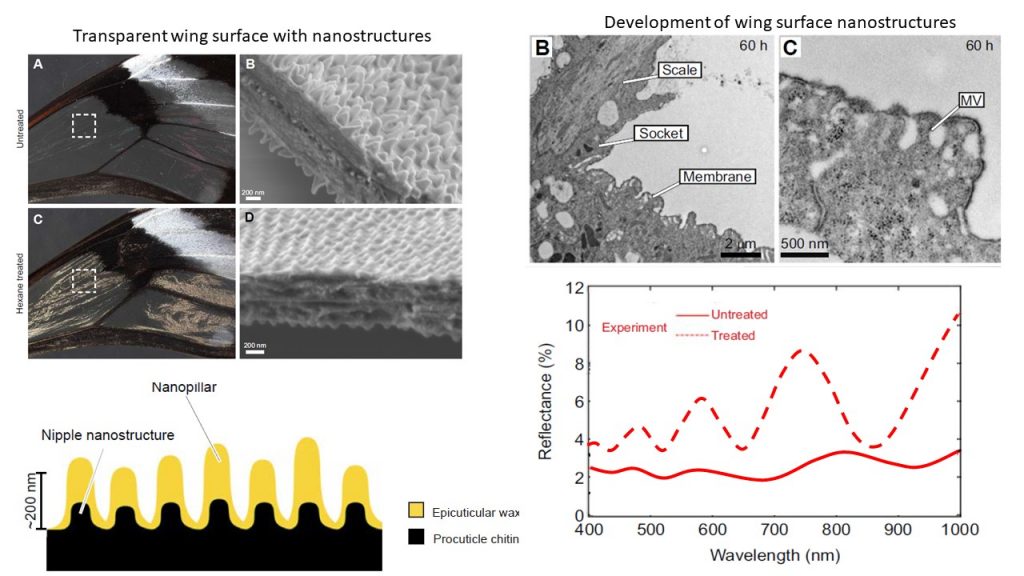
We also show that nanostructures on the wing membrane surface are composed of two layers: a lower layer of regularly arranged nipple-like nanostructures, and an upper layer of irregularly arranged wax-based nanopillars composed predominantly of long-chain n-alkanes. By chemically removing wax-based nanopillars, along with optical
spectroscopy and analytical simulations, we demonstrate that these nanopillars have antireflective properties and decrease surface reflections. Waxes have an intermediate refraction index (RI=1.34), which decreases the difference in refraction index seen by light beams striking the clearwing surface. They efficiently contribute – along with the low density of forked and monofid piliform cells – to increasing the level of transparency of wings in this species.
Evolution of micro and nanostructures
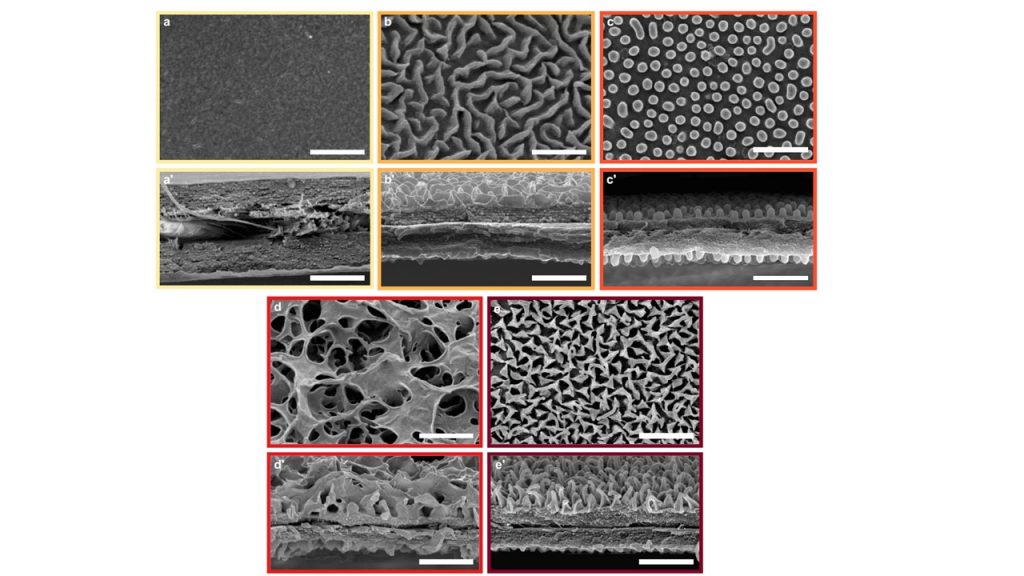
On the wing surface, nanostructures can be absent (a, a’), they can form mazes (b,b’), nipple arrays (c, c’), sponges (d, d’) or pillars (e, e’).
Studying the diversity of nanostructures in Ithomiini butterflies, we found nanostructure types that had not been described before (i.e. sponge) in any species (Pinna et al 2021). Important features that determine the efficiency of nanostructures at reducing surface reflections are the nanostructure type (pillars, nipples and sponges are more efficient), nanostructure density (denser nanostructures are more efficient), and nanostructure height (higher nanostructures are more efficient). Nanostructure types seem quite conserved at the subtribe level. For instance Godyridina have pillars while DIrcennina have sponge in general.
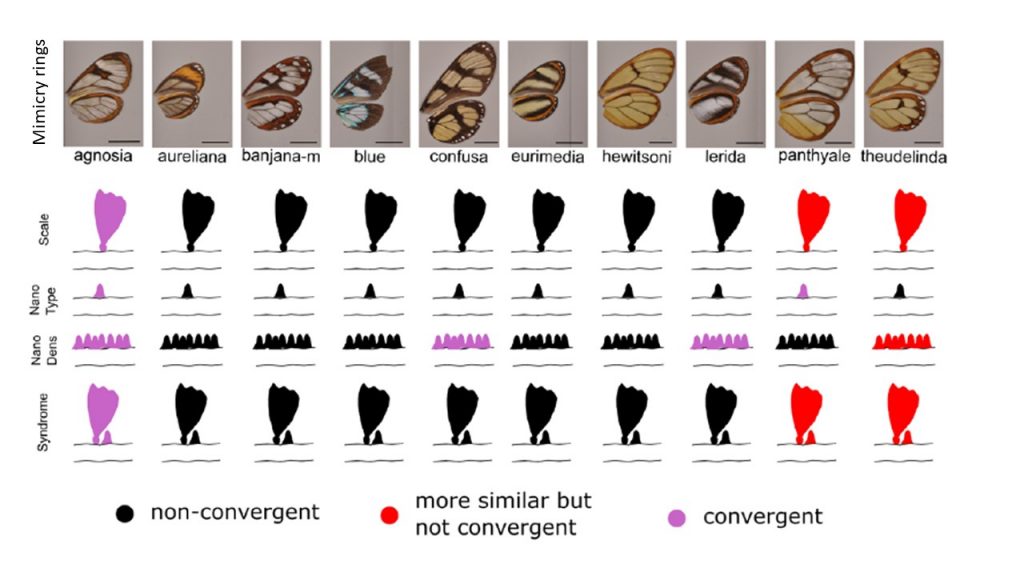
Ithomiini butterflies are involved in mimicry rings, where all co-mimics have been selected by visually-hunting predators to show a similar appearance. We wondered whether this extended to the type of structures involved in transparency (Pinna et al 2021). We tested whether structures were more similar than expected at random (similar, in red) and whether structures were more similar than expected at random given the phylogeny (convergent, in purple). When structures were not more similar than expected at random or given the phylogeny, structures were not convergent (in black). We found that few mimicry rings showed convergence even in structures (nanostructure density, nanostructure type or scales (erect coloured lamellar, erect coloured piliform, flat transparent lamellar). Although these rings are rare, they exist, showing that selection can act and foster convergence in structures underlying transparency between co-mimics.
What functions are fulfilled by transparent patches?
Optical properties
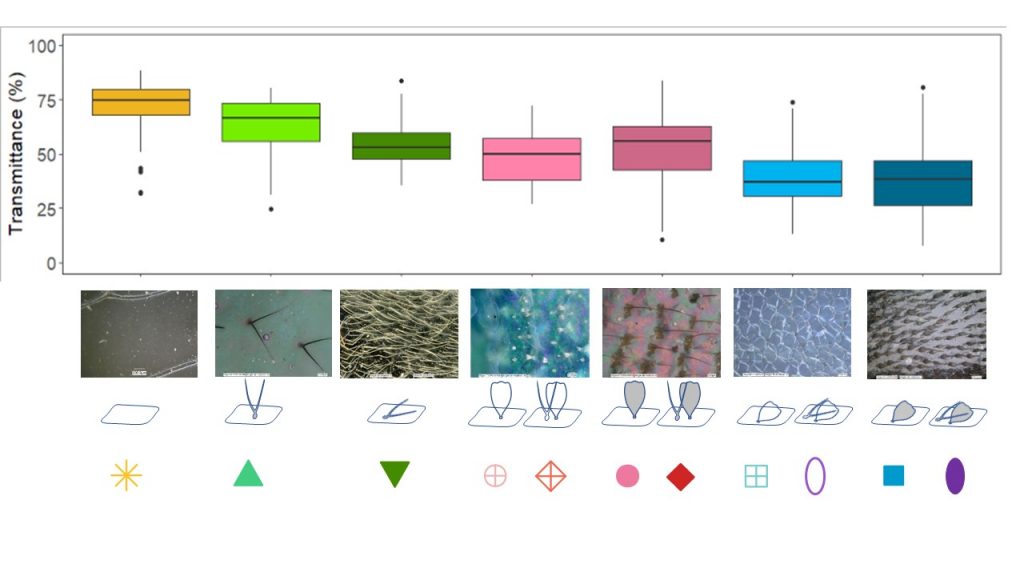
Measuring the transmission properties of the 123 species with transparent or translucent wings from these 31 families (Gomez et al 2021), we showed that microstructures influenced wing optical properties. The nude membrane transmits the most, erect scales transmit more light than flat scales, and transparent scales transmit more light than coloured scales. In total, the higher the coverage on the membrane (flat scales, coloured scales, scales in high density), the less light the membrane transmits. Nanostructures also contribute to increasing transmission, especially in species that are highly transparent.
Concealment
Does wing transparency in conspicuously-coloured butterflies decrease detectability by visually-hunting predators?
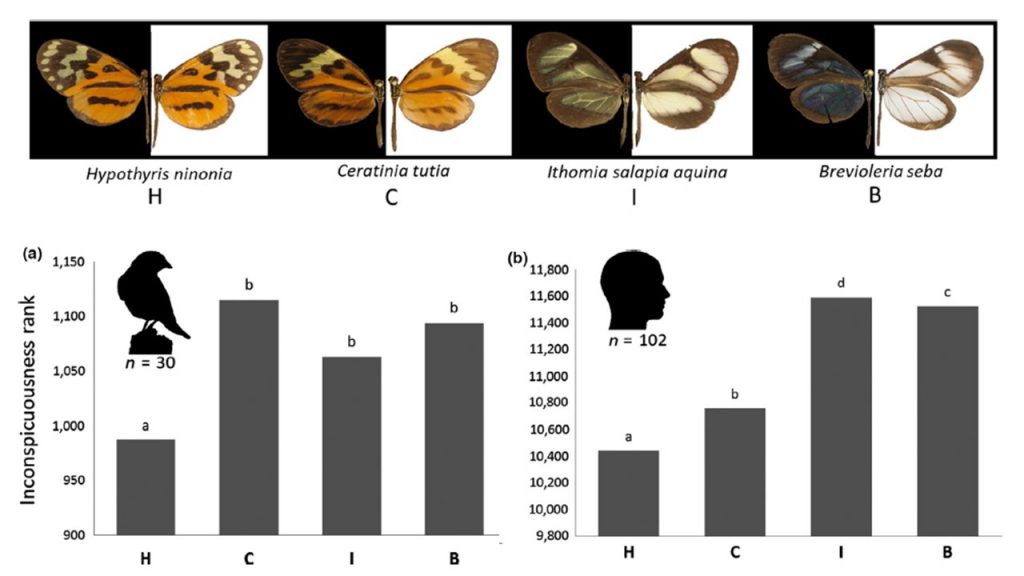
“inconspicuousness rank.” Butterfly transparency increases from left to right: Hypothyris ninonia (H), Ceratinia tutia (C), Ithomia salapia (I) and Brevioleria seba (B). All four species belong to the Ithomiini tribe.
Predation is an important selective pressure, and some prey have evolved conspicuous warning signals that advertise unpalatability (i.e., aposematism) as an antipredator defence. Conspicuous colour patterns have been shown effective as warning signals, by promoting predator learning and memory. Unexpectedly, some unpalatable butterfly species from the Ithomiini tribe possess transparent wings. We tested whether the transparency of butterfly wings was associated with a decreased detectability by predators, by comparing four butterfly species exhibiting different degrees of transparency, ranging from fully opaque to largely transparent (Arias et al 2019). We tested our prediction using both wild birds (a) and humans (b) in behavioural experiments. Vision modelling predicted butterfly detectability to be similar for these two predator types. In concordance with predictions, the most transparent species were almost never found first and were detected less often than the opaque species by both birds and humans, suggesting that transparency enhances crypsis. Our study demonstrates for the first time that transparency on land likely decreases detectability by visual predators.
Does wing transparency in cryptically-coloured butterflies provide increased protection against visually-hunting predators?
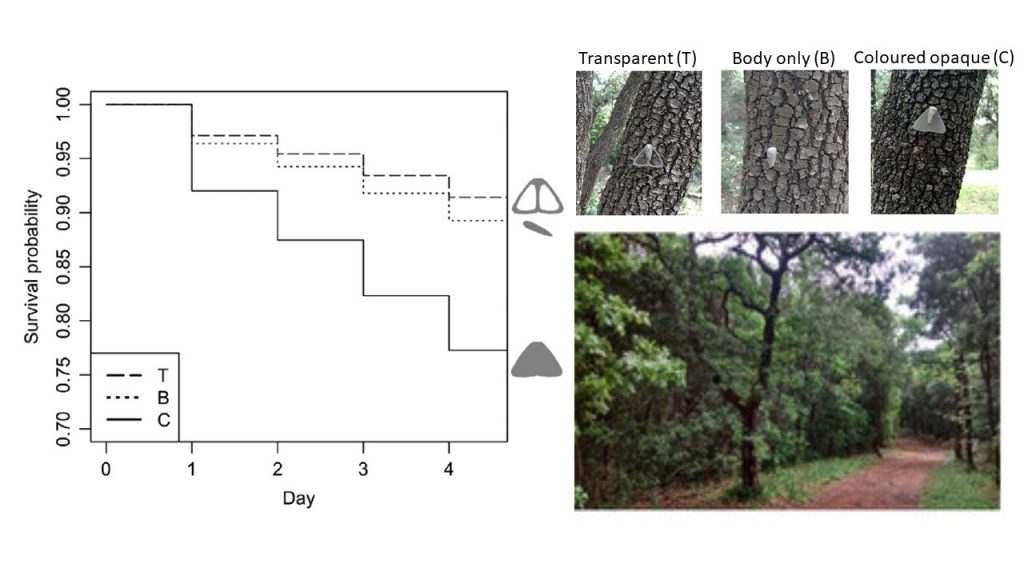
Does transparency help concealment from visually-hunting predators? This has been demonstrated for transparent species living in water but not for transparent species living on land. We placed artificial cryptic prey with and without transparent areas on trunks in a natural forest environment near Montpellier (Arias et al 2020). We then monitored their attacks by the local community of insectivorous birds. We found that prey with transparent areas were less attacked than uniformly-coloured cryptic prey, and as attacked as prey with only a body and no wings. This suggests that transparency enhances survival of transparent-coloured prey compared to opaque cryptically-coloured prey, likely due to increased concealment.
Does the size and location of transparent patches on the wings influence detectability by visually-hunting predators?
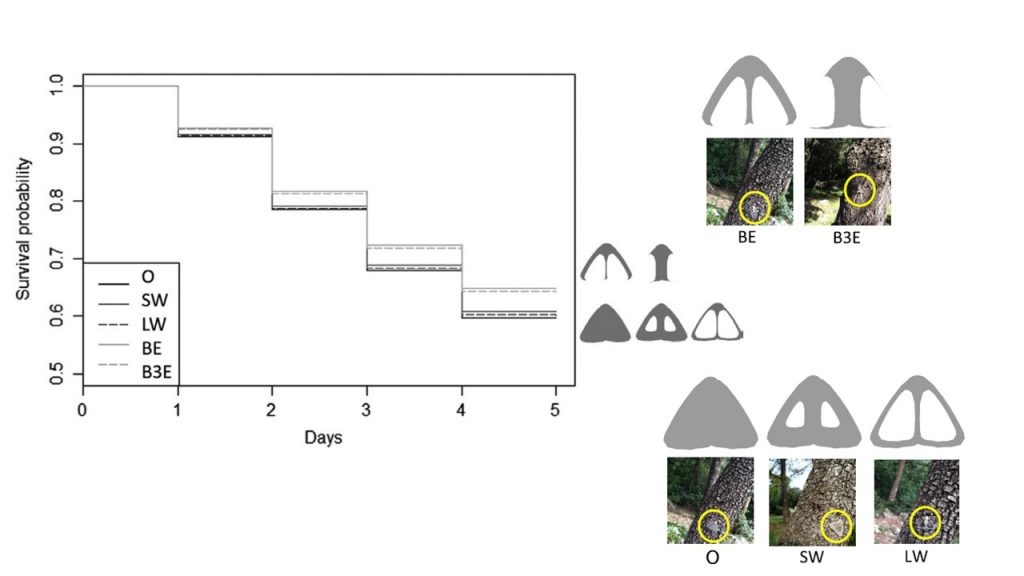
In opaque butterflies, disruptive colorations – in which colour patches appeared to disrupt the animal contour – are especially effective in decreasing prey detectability by visually-hunting predators. We wondered whether transparent elements could function in the same way. Using the same protocol as before, we tested how the size and position of transparent elements affect the predation of artificial moths by wild birds in the field (Arias et al 2021). Morphs with transparent elements touching wing borders showed a reduced predation risk, with the effect being the same regardless of the number of wing borders being touched. By contrast, transparent element size had little to no effect on predation risk. Overall, this experiment shows for the first time that transparency offers higher protection when it disrupts prey contour in terrestrial habitats.
Communication
Müllerian mimicry is a positive interspecific interaction, whereby co-occurring defended prey species share a common aposematic signal. Visually-hunting predators learn to avoid aposematic unpalatable prey and as they need to taste a few individuals, the risk of being eaten is diluted among all species living together and showing the same pattern, which is beneficial. In Lepidoptera, aposematic species typically harbour conspicuous opaque wing colour patterns with convergent optical properties among co-mimetic species. Surprisingly, some aposematic mimetic species have partially transparent wings, raising the questions of whether optical properties of transparent patches are also convergent. In other words, could transparency be involved in predator-prey communication?
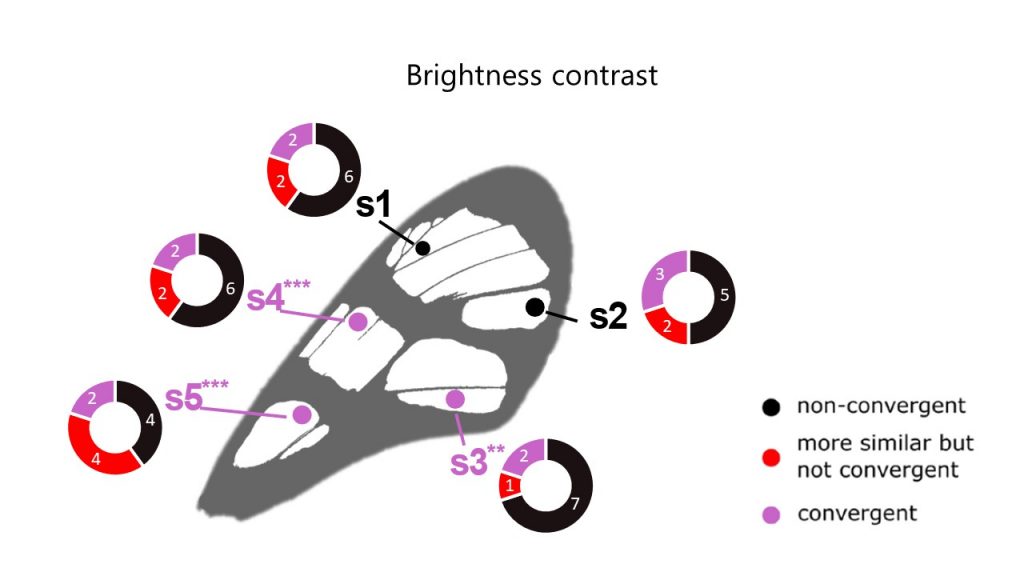
We conducted a comparative study of wing optics, micro and nanostructures in 62 Neotropical mimetic clearwing Lepidoptera belonging to 10 mimicry rings, using spectrophotometry and microscopy imaging (Pinna et al 2021). Using visual perception modelling, we computed the visual contrast between any two species as seen by a bird predator, for a particular point measured on the wing. We showed that transparency, as perceived by predators, is convergent among co-mimics in some mimicry rings. This shows that transparent patches converge in brightness but not in colour between co-mimics. Transparency is part of the aposematic signal and involved in communication.
How other functions classically fulfilled by scales are achieved in clearwing species?
In opaque species, scales typically fulfill multiple functions, such as communication, camouflage, thermoregulation, water repellency, flight enhancement, or resistance against pathogens. How are these functions fulfilled in transparent species?
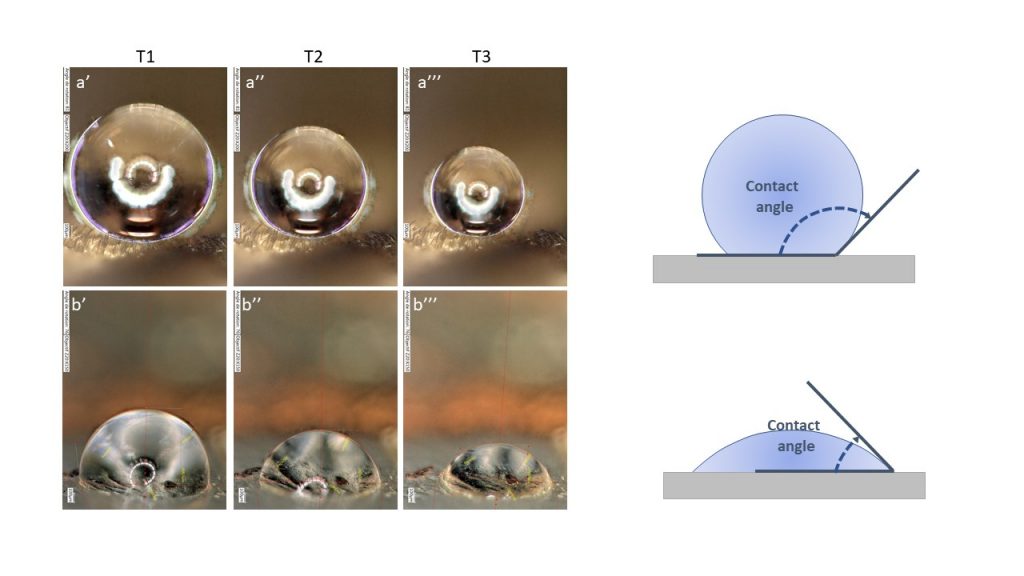
We considered 23 species showing the full range of diversity of structures (piliform and/or lamellar scales, flat or erect, coloured or transparent) and we tested wing water repellency in the opaque zone and in the transparent zone. We then analysed how contact angle varied according to microstructure (scale type, insertion, colour) and scale density.
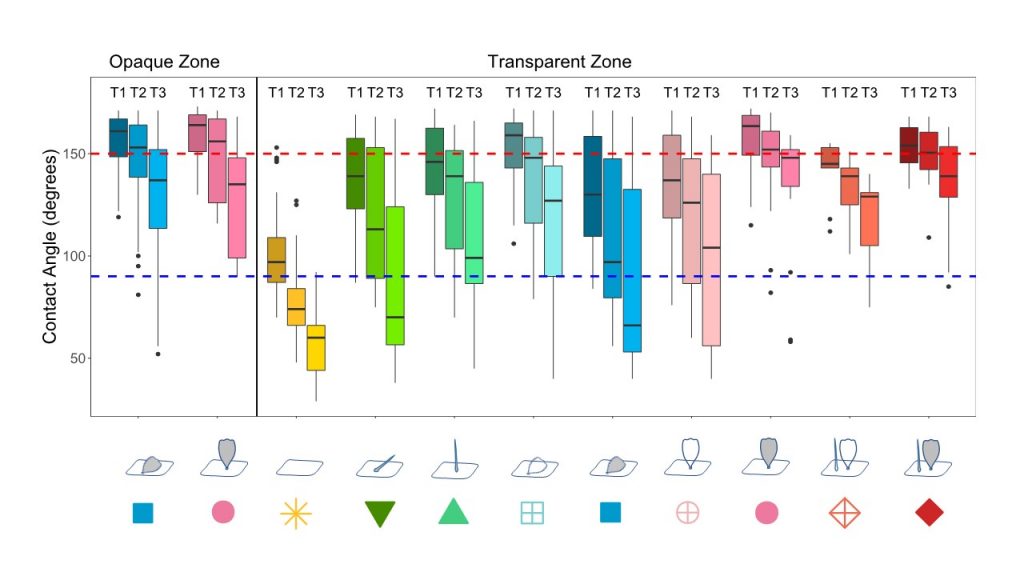
We found that hydrophobicity was lower in the transparent zone compared to the opaque zone, suggesting transparency was costly in terms of water repellency. In addition, we found that CA decreased with evaporation (going from T1 to T3), and more strongly in the transparent zone than in the opaque zone, which again pointed to a cost of transparency in case of tiny droplets. Structure played a role: a nude membrane was the least hydrophobic while combining two types of scales (piliform AND lamellar) resulted in a higher hydrophobicity and a lower loss of hydrophobicity with evaporation than having only one type of scales (piliform OR lamellar). Coloured scales showed a lower loss of hydrophobicity with evaporation than transparent scales of similar insertion and type.
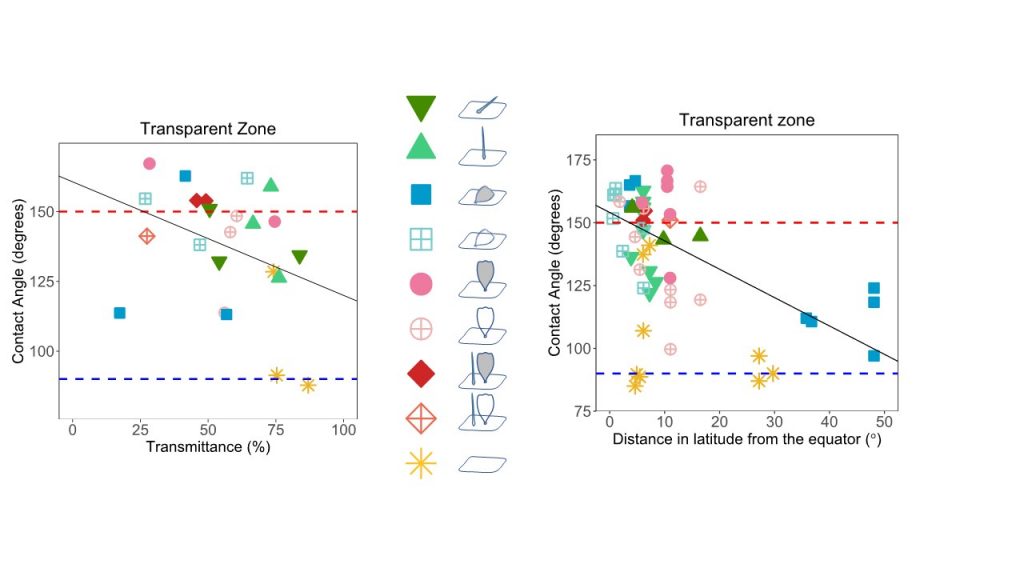
We found a trade-off between optical and hydrophobicity properties. Moreover, we found that tropical species showed a higher wing hydrophobicity in the transparent zone than temperate species, while they showed similar superhydrophobic opaque zones. Hence, structures that could maintain a high hydrophobicity while being transparent (like combined coloured erect piliform and lamellar scales) were favoured in the tropics where rainfall is high.